The first two experiments using the newly constructed collection of apparatus known as the Super BigBite Spectrometer were completed from Oct. 2021-Feb. 2022 in Jefferson Lab’s Experimental Hall A. Data were collected that will determine the neutron’s magnetic form factor (GMN) in a previously unexplored regime of momentum transfer Q2 up to 13.6 (GeV/c)2 with unprecedented precision. Form factor measurements at these energies are sensitive to the structure of the neutron at the sub-femtometer scale, and can resolve features of the neutron’s charge and current distributions at length scales approximately 20 times smaller than the size of a proton. These two completed experiments were the first in a family of precision studies of proton and neutron form factors at high momentum transfer using the SBS apparatus that will occupy the floor of Hall A through 2024. Professor Andrew Puckett’s group plays a leading role in the SBS collaboration (and the group is looking for several new graduate students to work on this exciting and high-impact program!).
Precision high momentum-transfer nucleon form factor measurements are extremely technically challenging, requiring several major innovations in detector technology and high-performance data acquisition and analysis. The GMN set of experiments achieved the first large-scale deployment and operation of Gas Electron Multiplier (GEMs) detectors in the high-luminosity, high-radiation, high-background-rate environment in Hall A. The GEMs were used in this set of experiments for tracking high-energy electrons through the BigBite Spectrometer, which was designed for detecting, tracking, and identifying scattered electrons with large angular and momentum acceptance at high luminosity. Given the large channel count and the high occupancy of the BigBite GEMs (approximately 42,000 readout strips with up to 30-40% of these firing in every triggered event), the SBS GMN run produced 2 petabytes of raw data (or typically about 1 GB/s during beam-on conditions). This is roughly 5 times as much raw data produced in four months of beam time in Hall A as the previous 25 years of Hall A running combined. Charged particle tracking in this extreme high-background environment is also extremely challenging, and UConn developed the software infrastructure and algorithms to do so with high performance and efficiency. The UConn group was one of the most actively involved in the preparation and execution of the experiment, developed the Monte Carlo simulation, event reconstruction and data analysis software, and is now leading the analysis of the collected data using Jefferson Lab’s scientific computing facilities. Two UConn Ph.D. students, Provakar Datta and Sebastian Seeds, will write their doctoral dissertations on the analysis of the SBS GMN dataset.

Figure 1 shows the collected Q^2 points for the extraction of GMN and the projected accuracy based on the data obtained, compared to existing data, selected theoretical models, and the projected Q2 coverage and precision of a measurement in Hall B with similar physics goals, but with larger systematic uncertainties from qualitatively different sources. The measurement of neutron form factors in the SBS-GMN experiment is based on the so-called “Ratio Method”, in which quasi-elastic electron-neutron and electron-proton scattering are measured simultaneously in scattering on a deuterium target (a deuterium nucleus is a weakly bound state of a single proton and a single neutron). By simultaneously detecting electron-neutron and electron-proton coincidence events in elastic kinematics, the ratio of electron-neutron and electron-proton scattering cross sections is determined with very small uncertainties. Combined with the existing knowledge of the electron-proton scattering cross section, the free neutron cross section can be extracted rather precisely.
To carry out this measurement, the SBS Collaboration constructed a large-acceptance Hadron Calorimeter (HCAL) consisting of large modules of many alternating layers of iron and plastic scintillator, which detects both protons and neutrons in the momentum range of these measurements with very high (and nearly identical) efficiencies, leading to much smaller systematic uncertainties compared to previous measurements of this type. Scattered electrons are detected in the BigBite spectrometer, and the scattering angles and momentum of the electron, as well as the location of the interaction vertex, are reconstructed from the precisely measured tracks of ionization they leave in the BigBite GEMs. Under the assumption of elastic scattering on quasi-free protons or neutrons, the scattered neutron or proton must carry all the energy and momentum transferred from the electron in the hard collision, allowing us to predict the location where the protons or neutrons should be detected in HCAL. To identify whether the scattering occurred on a proton or neutron, the scattered protons are given a small vertical deflection by the SBS dipole magnet so that they are well separated from the scattered neutrons by the time they are detected in HCAL.
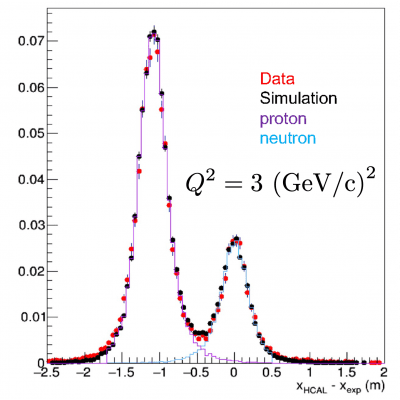
Figure 2 shows a comparison between real data from the SBS-GMN experiment obtained at Q2 = 3 GeV2 and the Monte Carlo simulation of the experiment, which includes the full details of the detector geometry and response, and the physics of quasi-elastic scattering of electrons by bound protons and neutrons in the liquid deuterium target, showing the clear separation between protons and neutrons based on magnetic deflection of the protons before they are detected by the SBS Hadron Calorimeter (HCAL), and the low level of background (at this Q2) from processes other than quasi-elastic scattering, demonstrating a very good understanding of the detector at such an early stage of the analysis.
The example event distributions shown below were obtained at an incident electron beam energy of 6 GeV and Q2 = 4.5 GeV2:
Figure 2 (above) shows the invariant mass distributions for reconstructed electrons in BigBite, from the hydrogen (left) and deuterium (right) targets, before and after applying cuts on the angle between the reconstructed momentum transfer direction and the reconstructed scattering angle of the nucleon (proton or neutron) detected in the SBS hadron calorimeter (HCAL). The hydrogen distribution shows a clear peak at the proton mass corresponding to elastic scattering, and the angular correlation cut removes most of the inelastic background, while keeping most of the events in the elastic proton peak. The deuterium distribution is “smeared” by the Fermi momentum of the bound nucleons in deuterium, and the distributions of events passing the angular correlation cut under the hypothesis that the detected nucleon is a proton (red) or neutron (blue) illustrate the relatively clean selection of quasi-elastic scattering and rejection of most inelastic events using the SBS dipole magnet and hadron calorimeter.
Figure 3 (above) illustrates the method for nucleon charge identification using the SBS dipole magnet and the SBS hadron calorimeter. The plot shows the difference in vertical position between the detected nucleon at HCAL and the expected position predicted from the reconstructed electron kinematics assuming elastic (or quasi-elastic) scattering. The distributions are shown for hydrogen and deuterium targets for three different SBS magnetic field settings (magnet off, 70% of maximum field, 100% of maximum field). The hydrogen distributions show a single peak corresponding to elastic electron-proton scattering, that moves as the SBS magnetic deflection is varied. The deuterium distribution with field off shows a single nucleon (proton plus neutron) peak, smeared by Fermi motion. The deuterium distributions with SBS field on show a clear separation into proton (deflected) and neutron (undeflected) peaks, with protons undergoing the same average deflection as seen with the hydrogen target.